Although most people started hearing about DNA* and mRNA** vaccines last year, these technologies have been developing for decades. In 1990, scientists started to explore how DNA and mRNA could be transferred to an organism to produce certain proteins*** [1], which led to the development of DNA and mRNA vaccines. As we explained in our post here. DNA and mRNA are types of genetic material. In a cell, DNA is transcribed into mRNA, which is then translated into proteins.
In the context of vaccination, we only need to know the genetic sequence of the pathogen to create a DNA or mRNA vaccine. Since scientists do not have to handle the pathogen itself but only its genetic sequence, these vaccines tend to be produced faster (more information here). Another advantage is that DNA and mRNA vaccines are more targeted because they only include the instructions for the antigen of interest (i.e., the protein that is meant to trigger the immune response and teach our body to fight the infection).
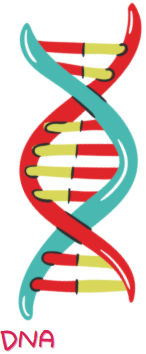
In the case of DNA vaccines, the DNA needs to enter inside the nucleus. This foreign DNA will remain as an extrachromosomal “plasmid” ****. In other words, it won’t be incorporated into our chromosomes*****, but it will remain floating in the nucleus as additional material. In a study where they injected DNA vaccines into the muscle, scientists discovered that the DNA does not significantly insert into our genome. In other words, the risk that the vaccine DNA integrates or alters our DNA is negligible [2]. Only certain methods of DNA delivery, like electroporation using electricity, are associated with a relatively increased risk [2].
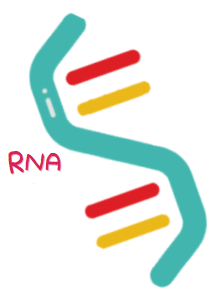
In the case of mRNA vaccines, that risk does not even exist. In our previous text, we discussed how our bodies naturally flow from DNA → RNA → protein, and humans do not have a mechanism to reverse this flow. Therefore, the mRNA in the vaccine will not be incorporated into our genome because it cannot be converted into DNA and it does not even get inside the nucleus.
When we compare a traditional vaccine based on proteins, versus an mRNA vaccine, versus a DNA vaccine, there are several differences [3]. Since DNA has the instructions to make mRNA, and mRNA has the instructions to build proteins, there is an amplification of the signal along the pathway. Thus, in theory, the “signal” should be more amplified in a DNA vaccine, followed by the mRNA vaccine and, in third place, a typical protein-based virus inactivated vaccine [3]. However, this amplification does not necessarily imply a higher immune response.
In clinical trials, it appears that some DNA vaccines evoke an immune response but it may not be sufficiently strong to show clear benefits (this statement refers to DNA vaccines in general; however, some DNA vaccines for COVID-19 are currently being developed and evaluated [4]). More research is needed on how to optimize DNA vaccines to enhance immune response and their impact on society. Thus, scientists are devising alternatives to prevent DNA degradation, facilitate its entry into the nucleus, and increase the delivery of the DNA into targeted cells [5].
In contrast, as we mentioned before, an mRNA vaccine has an advantage: it does not need to enter the nucleus. Indeed, mRNA is already one step closer to producing the protein and generating an immune response. Moreover, an mRNA vaccine stimulates the immune system much more than a DNA vaccine. However, mRNA is relatively unstable, and some drugs could affect its processing [3].
In general, DNA and mRNA vaccines have been explored and researched for several years. Currently, mRNA vaccines have been particularly useful in preventing COVID-19, and they have opened up new lines of research. Since many people are concerned about their genetic information being changed, it is important to remember that mRNA vaccines do not change our genome. Indeed, in the human body, mRNA cannot naturally transform into DNA, so it does not enter the nucleus nor integrate into our chromosomes.
*DNA - deoxyribonucleic acid, one of the nucleic acids found in living cells. DNA is formed by 4 nucleotides, which can be organized in different sequences and different lengths like letters are organized to form a text. In this case, the “text” formed by the sequence contains the instructions to form a whole organism. DNA is normally found as a paired double strand, in a double helix shape.
**RNA - Ribonucleic acid, one of the nucleic acids found in cells. It is transcribed from DNA. There are many types of RNAs, some of which can have a specific function controlling or regulating some processes in cells while others (known as messenger RNAs, or mRNAs) carry the genetic information that can be translated into proteins by ribosomes.
***Proteins - a molecule that forms the structure and gives function to organisms at the most basic level. Proteins consist of amino acids, which can be combined in different sequences to form different protein structures with different functions in cells.
****Plasmid - Small circular DNA molecule separated from the chromosomal DNA. They are mainly found in bacteria and typically include a gene that offers some advantages for survival, such as antibiotic resistance.
*****Chromosome - a long DNA molecule that can be compacted into smaller and denser structures inside cells. This compacting action is made possible by the assistance of some specific proteins. Chromosomes contain all or part of the genetic material of an organism. Humans usually have 46 chromosomes per cell.
________________________
Written by: Nicole
Edited by: María and Natasha
BioDecoded is a volunteer group committed to sharing accurate scientific information. If you have any questions about this topic or would like to learn more, please send us your questions on the “contact” page or our email.
References:
Wolff, J. A., Malone, R. W., Williams, P., Chong, W., Acsadi, G., Jani, A., & Felgner, P. L. (1990). Direct gene transfer into mouse muscle in vivo. Science (New York, N.Y.), 247(4949 Pt 1), 1465–1468. https://doi.org/10.1126/science.1690918
Wang, Z., Troilo, P. J., Wang, X., Griffiths, T. G., Pacchione, S. J., Barnum, A. B., Harper, L. B., Pauley, C. J., Niu, Z., Denisova, L., Follmer, T. T., Rizzuto, G., Ciliberto, G., Fattori, E., Monica, N. L., Manam, S., & Ledwith, B. J. (2004). Detection of integration of plasmid DNA into host genomic DNA following intramuscular injection and electroporation. Gene therapy, 11(8), 711–721. https://doi.org/10.1038/sj.gt.3302213
Liu M. A. (2019). A Comparison of Plasmid DNA and mRNA as Vaccine Technologies. Vaccines, 7(2), 37. https://doi.org/10.3390/vaccines7020037
Silveira, M. M., Moreira, G., & Mendonça, M. (2021). DNA vaccines against COVID-19: Perspectives and challenges. Life sciences, 267, 118919. https://doi.org/10.1016/j.lfs.2020.118919
Hobernik, D., & Bros, M. (2018). DNA Vaccines-How Far From Clinical Use?. International journal of molecular sciences, 19(11), 3605. https://doi.org/10.3390/ijms19113605
コメント